Small Volume Detection
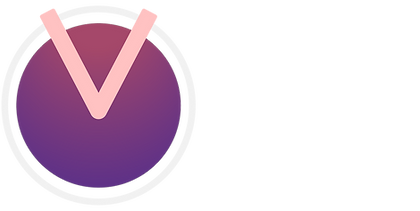

The considerable advantages (with respect to speed, throughput, analytical performance, selectivity, automation and control) that are afforded through the use of microfluidic systems are in large part made possible by system downscaling and the associated improvements in mass and thermal transfer. Nonetheless, handling and processing fluids with instantaneous volumes on the femtoliter-to-nanoliter scale represents a critical challenge for molecular detection, and still defines one of the key limitations in the use of a microfluidic system in a given application. That is to say, although system downsizing leads to significant improvements in analytical performance, such gains are offset by a progressive reduction in the number of molecules which must be detected to report the chemical or biological state of the system. For example, it is well-recognized that the transfer of capillary electrophoresis to planar chip formats allows for ultra-short analysis times and high numbers of theoretical plates ☍ ☍. However, when performing electrophoretic separations within chip-based formats, analyte injection volumes are commonly no larger than 50 pL. This means that for an analyte concentration of 1 nM, only about 3000 molecules are available for separation and subsequent detection.
Over the past twenty years, we and others have addressed the issue of small volume analysis through the development of a range of detection methodologies. These include fluorescence and absorption spectroscopies, refractive index variation, Raman spectroscopies, infra-red spectroscopy, chemiluminescence, thermal lens microscopy and mass spectrometry. The pervasive adoption of optical methods is unsurprising, since chip-based microfluidic systems are typically fabricated from glasses, polymers and plastics, which have excellent optical transmission characteristics in the ultraviolet, visible and near-infrared regions of the electromagnetic spectrum. Accordingly, molecular samples can be non-invasively probed via the absorption, emission or scattering of radiation.
Although the above methods are efficient at assaying small sample volumes on short timescales, they are typically inefficient at extracting the enormous amounts of chemical and biological information provided by microfluidic systems. Put simply, only a small fraction of the available information is commonly harvested during the detection process. To this end, a central theme of our activities in the area of small volume detection has been the development of novel optical methods that are able to perform multi-parameter analysis in a rapid and efficient manner. For example, we have pioneered the development of fluorescence lifetime imaging methods to probe temperature, pH, binding, viscosity and molecule concentration with unrivalled temporal and spatial precision ☍ ☍ ☍. We have also developed a range of vibrational and photothermal techniques for high sensitivity molecular fingerprinting ☍ ☍. These are of particular significance to chemical and biological systems, since they directly engender the high-throughput and sensitive analysis of non-fluorescent species, including small molecules, ions, biomolecule and nanomaterials. Most recently, we have developed entirely new ways of imaging rapidly moving species within microfluidic flows. These methods leverage stroboscopic illumination and allow the performance of enormous numbers of experiments on short timescales. Notable examples include the precision screening of over 1500 discrete enzymatic assays within 10 seconds ☍, and the demonstration of high-resolution multi-parametric imaging flow cytometry at unprecedented throughputs (in excess of 100,000 cells per second) ☍.